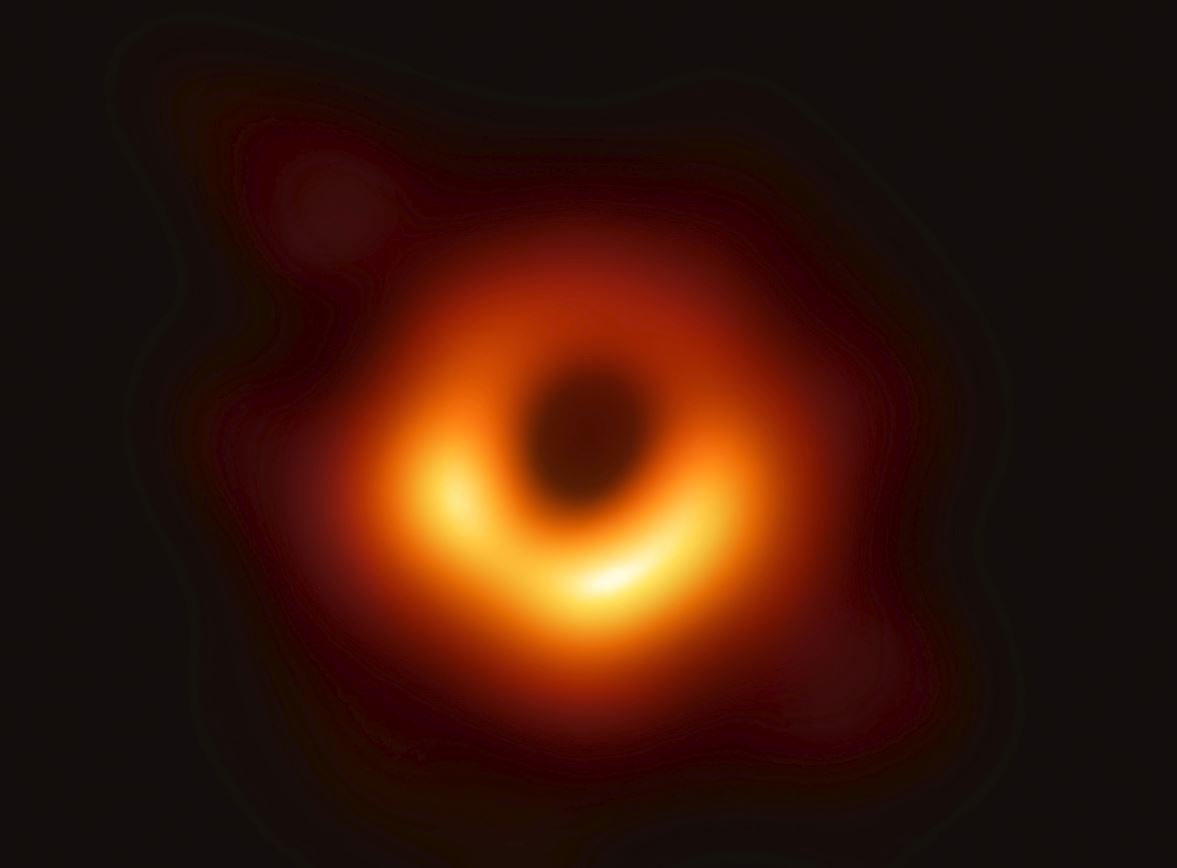
Convincing Einstein that Black Holes are physical objects out there!
Dr Sheldon Cooper and friends will be thrilled at the release of the black hole image. The popular television series ‘Big Bang Theory’s’ physicists, who represent the quantum mechanics and physics researchers in a lighter vein are often found quarrelling about testing theoretical physics. A team of scientists captured the image of the Black Hole Sagittarius A at the centre of the galaxy milky way on April 10. This long-sought image provides the strongest evidence to date for the existence of super-massive black holes and opens a new window onto the study of black holes, their event horizons, and gravity.
The Federal spoke to Dr Birjoo Vaishnav, Fellow of Radiation Oncology Physics at Mayo Clinic, Minnesota, United States on what this means and why it is historic. He specialises in Black hole theory research and Relativistic Astrophysics.
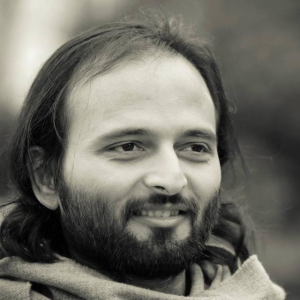
How did the scientists capture this image?
Scientists assembled a coalition called Event Horizon Telescope (EHT) to capture the first image of a black hole. The EHT used an array of eight radio telescopes from six stations across the Earth, including one at Antarctica. Radio waves at the wavelength of 1.3 mm were chosen as they could penetrate through the interstellar medium and hence reveal the features of the areas closest to the event horizon of the black hole. Such radio waves are emitted by electrons falling into the black hole. In this case the black hole is a supermassive one that is its mass is billions of times that of the sun. As a result the emissions are in radio frequency. This black hole is also known to be an Active Galactic Nucleus, with strong activity of radio jets resulting from the way the plasma moves around the black hole.
The Innermost activity of the plasma could not be resolved by single telescopes. Imagine a very large dish antenna – the size of the Earth. That is the size of the dish required to capture radio waves from galaxies far, far away. Combining the signals from many dish antennas to get a longer baseline is called VLBI Very Large Baseline Interferometry. Using the signals of eight such VLBI instruments, each consisting of several dishes, was what enabled this discovery.
Scientists collected enormous amounts of data, about several petabytes of data per day, which could not be shared across the internet. The data had to be shipped to a common location on physical hard disks to obtain an image by processing the data from it. The data was taken over five days in April 2017.
Why is this important?
It is a huge feat because this is the first direct observation of the areas closest to the event horizon of a black hole. For more than a century, the evidence for black holes has been indirect. The image that was released yesterday (April 10), is the result of processing the data collected in 2017. The scientists had also detected the gravitational waves from collision of two black holes in 2016 years ago, but that was for black holes ten to hundred times the mass of the sun. This image is for a black hole about seven billion times the mass of the sun, about thousand times more massive than the one at the center of Milky Way.
The measurements provide a stringent test of Einstein’s general relativity in the strong field regime.
Why has it taken the scientists so long if telescopes have been around for a while now?
Just like how mobile-phones we’re hardly so sophisticated two decades ago, the technology required to combine such large amounts of data from multiple radio telescopes had to be developed over two decades of collaborative effort of the EHT consortium. With technological advancements, scientists were able to develop algorithms to calibrate, model, test, combine and decipher these signals. The timing of each of the signal had to be accurately noted by an atomic clock. Due to different telescopes being at different location on earth, there would be difference in the timing that needed to be accounted for depending on the relative location. Complex simulation of the magnetic field and the motion of electrons around the black holes had to be made in order to be able to compare the theory to the measurements. All of this required enormous computational capacity which did not exist thirty years ago.
An average smartphone of today has more capacity than the satellite and technology that the NASA used in the 70s to send humans into space.
Why do black holes ‘suck’ things into them? Will they also suck our solar system, someday?
Black holes are not like vacuum cleaners that suck anything that comes near them. Just as the moon or an apple or a basketball is pulled to the earth, stars, ions and other objects are pulled toward black holes due to its gravitational force. This gravitational pull is like that of any other star. The only difference between them is light-when earth is pulled by the sun, enabling it to go around in an orbit, we are also able to see its light. Instead if someone replaced the sun with a black hole of the same mass, the only difference will be we may not see much light coming from it. The light around black hole is due to the matter emitting radiation before it falls in. Just like how particles going straight toward the sun would fall into it due to its gravitational force, particles fall (they don’t get sucked) into black holes, as they approach it if they get too close.
Most galaxies are believed to have a massive (million to billion solar mass) black hole at its centre. But as we saw above, unless for some reason the solar system decides to head straight into the one at the center of the Milky Way, there is no chance of it “getting sucked”. Even if we decide to head straight into the black hole at the center of our galaxy, it would take billions of years to get there.
Indian astrophysicist Subrahmanyan Chandrasekhar in the early 1920s worked out that the mass of a star had to be at the minimum 1.4 times the mass of the sun to form a black hole. In 1984 he was awarded Nobel Prize in physics for his extensive work on understanding the astrophysics of black holes, white dwarfs and neutron stars and till date the 1.4 solar mass limit is called Chandrashekhar Mass limit. After almost a century, yesterday’s image proves great minds like him right. These black holes emit certain ‘frequencies’ or radio waves. The smaller the black hole, the higher the radiation is.
How will this alter Quantum Mechanics or Physics as we know it?
The theory of Quantum Mechanics historically evolved by scientists close observation of the spectrum of sunlight and the structure of it. Science evolves by close interplay of theoretical predictions and experiments and observation. Nobody in the nineteenth century could have predicted that a refined study of optics and spectra would lead to our understanding of the atomic structure and quantum mechanics one day. Similarly having access to direct observations near the event horizon of black holes is a first step in a journey toward understanding the possible limitations of our present understanding of gravity and general relativity. It is possible that this could in few decades help us rule out some of the fancier efforts like string theory which seek to model the quantum nature of gravity itself.
Interestingly, Einstein for a while had bet against the existence of black holes. He would have been pleasantly surprised to know that even when he might have lost the bet, his theory and ideas would have won yet again.