- Home
- IPL 2025
- The Great Language Divide
- News
- Premium
- THE FEDERAL SPECIAL
- Analysis
- States
- Perspective
- Videos
- Education
- Entertainment
- Elections
- Features
- Health
- Business
- Series
- Bishnoi's Men
- NEET TANGLE
- Economy Series
- Earth Day
- Kashmir’s Frozen Turbulence
- India@75
- The legend of Ramjanmabhoomi
- Liberalisation@30
- How to tame a dragon
- Celebrating biodiversity
- Farm Matters
- 50 days of solitude
- Bringing Migrants Home
- Budget 2020
- Jharkhand Votes
- The Federal Investigates
- The Federal Impact
- Vanishing Sand
- Gandhi @ 150
- Andhra Today
- Field report
- Operation Gulmarg
- Pandemic @1 Mn in India
- The Federal Year-End
- The Zero Year
- Science
- Brand studio
- Newsletter
- Elections 2024
- Home
- Delimitation Debate
- IPL 2025
- NewsNews
- Analysis
- StatesStates
- PerspectivePerspective
- VideosVideos
- Education
- Entertainment
- ElectionsElections
- Features
- Health
- BusinessBusiness
- Premium
- Loading...
Premium
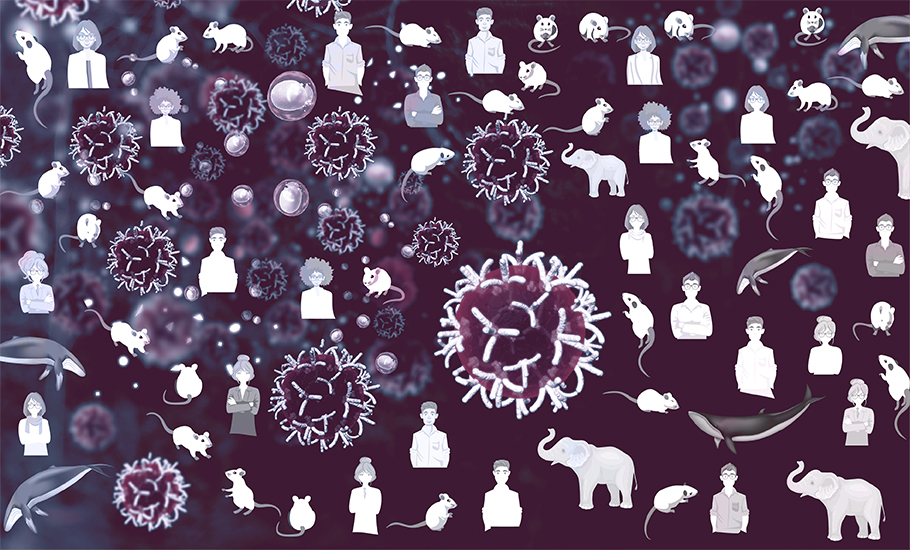
Why mice and humans are more likely to die of cancer than elephants
As the cell divides in our body and produces two new daughter cells, errors may sometimes creep into the replicated DNA. A tiny little fraction of these errors, called mutations, could lead to cancer, making the cells multiply uncontrolled. A mouse lives for about a year and has nearly 40 billion cells. A dog with trillion cells lives for 10-13 years. An adult human has about 37 trillion...
As the cell divides in our body and produces two new daughter cells, errors may sometimes creep into the replicated DNA. A tiny little fraction of these errors, called mutations, could lead to cancer, making the cells multiply uncontrolled.
A mouse lives for about a year and has nearly 40 billion cells. A dog with trillion cells lives for 10-13 years. An adult human has about 37 trillion cells and a lifespan of about 80. An elephant has about a hundred times more cells than a human and lives nearly 70 years. A Blue whale has whooping 100 quadrillions and endures for almost a hundred years.
When we have two playing cards in our hand, the odds of one of them being A spade are slim. However, when we have the whole pack of 53 cards, one of them is inevitably A spade. As the number of cards, we hold to increase the odds that one of them being A spade, escalate. Alternatively, assume we draw two cards multiple times, at least once, one of them being A spade hike with the number of turns increasing. Therefore, naturally longer we live and the more the number of replicating cells in our body, there must be greater the chance of cancer-causing mutations.
Consequently, a human with about 100 times more cells and living longer must have a greater chance of cancerous mutation than a mouse. By the same logic, cancer must be like a common cold for an elephant with 100 times more cells than a human. Whales have trillions more cells than humans. If the number of cells determines the risk, most of them should die of cancer even before adulthood. Curiously, the elephants and whales not only survive to old age but have a meagre rate of cancer.
Called the Peto’s paradox, the absence of correlation between cell numbers and longevity to cancer incidence in mammals is a biological enigma. An innovative study led by Alex Cagan, a staff scientist at the Wellcome Sanger Institute in England, has shown that the relationship between lifespan and mutation rates in different species partially explains the puzzle.
Cell division
We were born at the instant when the sperm from the father fertilised the egg from the mother. The first cell divides through cell division to become two daughter cells. The parent cell first makes sufficient copies of cellular machinery, like cell organelles, and makes two identical copies of the DNA. These are neatly divided between the two daughter cells. These two cells divide to become four, and then eight, and so to become about 12,50,00,00,00,000 (1.25 trillion) cells that the newborn has. During this process, the cell division must have been around 41 times. As the infant grows and becomes an adult, many more cells are added. An average 30-year-old adult weighing about 70 kilos has 30 trillion cells. The growth is possible only when the cells divide and produce new daughter cells.
In addition, cells also divide to heal a wound and replace worn-out cells. When we accidentally bite our lip or cut a finger, the wound heals in a matter of days. New cells grow to repair the damaged cells. The existing skin cells divide and produce new cells to repair the injury.
Cells divide many times but not indefinitely. Cells, too, are worn out, like a used bicycle over time. As the cells age, at one point, they stop dividing and die. Typically the cells in the heart, eyes and brain last almost a lifetime; muscle cells endure for about 30 to 70 years. Nonetheless, most of the cells in our body last from a few days to a few months; white blood cells only live for about 13 days, red blood cells for 120 days, and liver cells for 18 months. These cells are replaced by cell division.
Cancer
About 5%–10% of all cancers are inherited or hereditary. It is transmitted by either of the parents through the mutated gene in the sperm or an egg cell. The most common cause of cancer is mutations in the somatic cells. These cells replicate and proliferate during growth, repair or routine replacement.
About 330 billion cells are rejuvenated daily in a human adult, either for the growth of the human body or as a replacement for damaged or old cells. This is whooping one per cent of all human cells. This means in about four months, almost all body cells are replaced with new ones. In each cellular division, parent cells make two copies of their entire genome to be passed on to the two daughter cells. Unavoidably, a few mistakes creep in during the replication process when the DNA copying mechanisms fail to faithfully duplicate the genetic code. Most of these mutations are harmless. They may not cause a severe dent in the function of the cell. However, suppose the mutation occurs in critical areas of the genome. In that case, it can impair the cell’s normal functioning or make it cancerous. Tobacco use and environmental factors often exacerbate mutations, thus increasing the chance of cancer.
Fidelity of the genomic information is crucial, and hence there are many layers of genomic housekeeping mechanism. The DNA repair genes and error correction gene at the first level rectify the mutation. If it gets past and flawed cells are formed, they are induced to self-destruct.
Cancer does not arise from one single unfortunate mutation. The prevailing hypothesis posits a cascade of mutations occurring in the same set of cells in subsequent cellular division. First, the regulation that controls cell growth is impaired. That mutant cell, again by accident, acquires a mutation that permits proliferation. A third mutation deactivates the mechanism that induces the self-destruction of damaged cells. As mutations accumulate, the tumour becomes malignant.
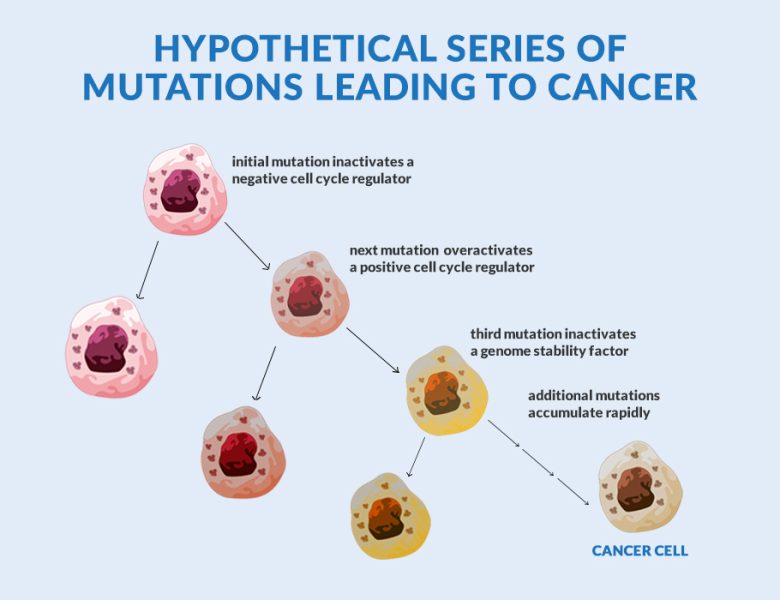
Usually, cells have regulatory genes that promote cell division and another set of genes that instruct old and damaged cells to perish. Mutations may impair the working of the regulatory genes letting the old and abnormal cells resist death. Complementing this, another mutation may allow the genes that encourage cellular division to stay in the ‘on’ position. With not many cells dropping dead, the new cells would be crowding and jostling for space. A lump called a tumour is formed. Most of the time, the tumours are like common skin warts that remain confined to their original location. However, when the tumour cells are malignant, they edge out normal cells, invade the surrounding tissue, and spread throughout the body via the circulatory or lymphatic systems. A malignant tumour is a cancer.
Peto’s paradox
Experimental studies showed that all the cells in the tumour are produced by one single mutant cell. Further, epidemiological studies show that cancer is an age-associated disease, where old individuals are more prone to develop the disease. Moreover, body size and height are positively correlated with cancer incidence. This means that the number of cells influences the possibility of cancer in humans. In a nutshell, within the human species, the cancer risk is elevated with age and body mass, as one would expect.
When the number of cells is higher, or the organism lives longer, then the chance of emergence of a mutant cell must be higher. However, it appears that is not the case when comparing animals. In 1977, the cancer epidemiologist Richard Peto compared the cancer rates in humans and mice. He noted that a man has 1,000 times as many cells as a mouse…. and we usually live at least 30 times as long as mice… However, it seems that, in the wild, the probabilities of carcinoma induction in mice and in men are not vastly different. He wondered, “Are our stem cells really, then, 1 billion or 1 trillion times more “cancer-proof” than murine stem cells?… If human DNA is no more resistant to mutagenesis in vitro than mouse DNA, why don’t we all die of notable carcinomas at an early age?”
Humans witness 10,000 times more cell division in their lifespan than mice, yet both face almost the same cancer risk. Just 4.8 per cent of elephants die of cancer. However, death due to human cancer is around 22% in the USA, with high life expectancy and about 6% in India. Surprisingly, Blue whales, with approximately 3,000 times more cells than humans, appear to be not inflicted by cancer. Dubbed the Peto’s paradox, this perplexing biological fact is an evolutionary conundrum that has puzzled the scientific community since then.
Cancer incidence in mammals
Epidemiological studies on the human population give us a glimpse into the cancer incident rates. Comparing the population of smokers and non-smokers, we can eliminate the contribution of factors such as tobacco to estimate cancer incidents among humans. How do we know the incidence of cancer among elephants or whales?
Species360, a non-profit organisation, has collected zoo and aquarium data from about 1,200 zoos worldwide. Ploughing through this data, researchers Orsolya Vincze of the University of Montpellier, CNRS, IRD, Montpellier, France, computed the cancer risk among various mammals. The Zoological Information Management System (ZIMS) had records of 110,148 animals belonging to 191 species, including data on their age, sex and other details. The postmortem reports of 11,840 individual animals pinpointed the cause of death of these animals in captivity. The researchers computed the cancer mortality rate for each species from these data.
In the study, published in a leading international science journal Nature (January 2022), researchers found that most of the species carried some cancer risk, with two exceptions. None of the Blackbuck (a kind of antelope) and the Patagonian mara (a type of rodent) captive in the zoo seemed to have died of cancer. The cancer mortality rate is as high as 57.14% in Dasyuroides byrnei, called Kowari, a brushy-tailed marsupial rat native to Australia. Further, about 25% of Carnivora, like clouded leopards, Bat-eared foxes and Red wolfs, die of cancer. In 41 species, the study found that the cancer mortality rate exceeded 10%. Surprisingly, the researchers found that the animal size is not correlated to the cancer mortality risk of the species. This implied some unknown natural anticancer mechanisms helped the long-living animals and animals with considerable cells to beat back cancer-causing mutations.
Mutation rates and life span
Is the rate of mutation constant in young and old? Does the mutation rate vary between small and large animals? Does the lifespan of the species have any correlation to the mutation rate? Alex Cagan explored these questions and published their surprising findings in Nature (April 2022).
The researchers collected DNA from the crypts, tiny folds found in the small intestines and colon lining. All the cells in each crypt have descended from a single stem cell. This means they all must be clones of each other, except for mutations.
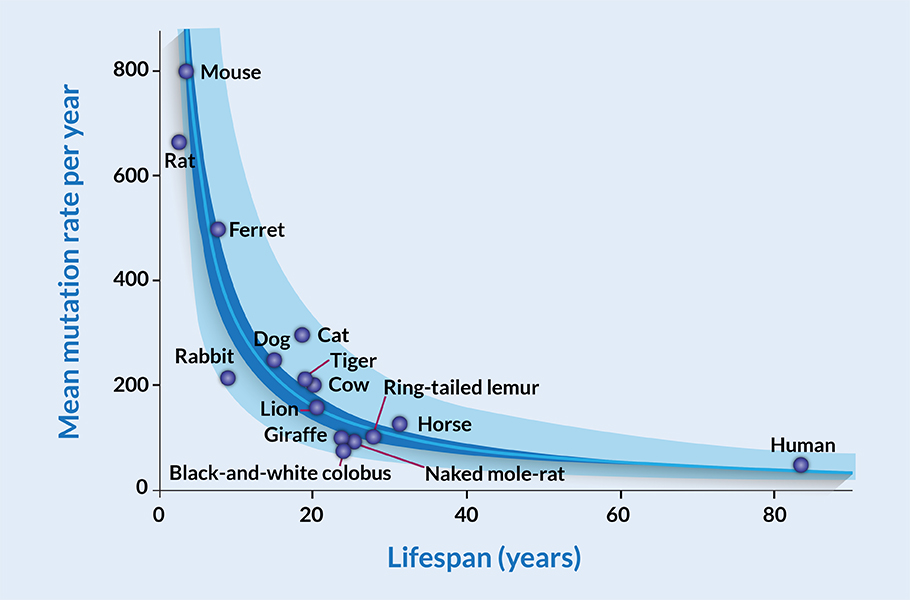
The samples were collected from 16 species, including humans, black-and-white colobus monkeys, cats, cows, dogs, ferrets, giraffes, harbor porpoises, horses, lions, mice, naked mole rats, rabbits, rats, ring-tailed lemurs and tigers. Among these animals, humans had the most extended lifespan of about 80, and mice and rats had the shortest lifespan of around 3.7 years. The DNA from each of these samples was sequenced. By comparing the DNA sequence, the researchers counted the mutation rate of each individual animal. By taking into account the age of that animal and the mutations found in the crypt cells, the mutation rate was determined.
In humans, an earlier study had shown that crypt cells pick up mutations at a constant rate as the person ages. What about the other animals? The speed of the mutation may be low when the animal is young, and the rates go high as the animal age. The researchers compared the total number of mutations in individuals of different ages. For example, they correlated the number of mutations in one-year-old mice to mutations in two-year-old mice.
The results astonished the researchers. Mutations accumulated at nearly a constant rate throughout the lifespan of the animals. On the other hand, mutation rates of one animal varied from others. For instance, on the average human crypt cells accumulated 47 mutations each year; in mice, it was a whopping 796 per year. The researchers found that dogs have around 249 annual mutations while lions have 160. They compared the mutation rate with the lifespan of animals. The typical lifespan of mice, dogs, lions and humans is about 3.7, 13, 20 and 80 years, respectively.
The writing on the wall was clear; the mutation rate of a species is negatively correlated with its lifespan. The shorter the lifespan, the more mutations it amasses; the longer the lifespan, the species accumulate fewer mutations per year.
Puzzlingly, there was no association between mutation rates and the typical body mass of the species. The mutation rates of Giraffe and Naked mole-rat are 99 and 93, respectively, with a similar lifespan of 24 and 25 years. However, the average Giraffe is 23,000 times more body mass than a Naked adult mole-rat. It did not matter if the species was tiny or enormous; the rate of mutation was strongly linked to only its lifespan. This surprisingly implies that despite variations in size, and lifespan, mammals end their life with nearly the same number of mutations at the end of their biological lifespan. This number is around 3,200 mutations.
Mutational clock
This study does not explain why the long-lived animals’ mutational clocks tick slower than short-lived animals. However, one research led by Jan Vijg, a professor and chair of the Department of Genetics at the Albert Einstein College of Medicine, Bronx, NY, suggests an explanation.
In this study published in the journal Science Advances (October 2021), the scientists took samples of fibroblasts, a type of cell found in connective tissue, from the lungs of mice, guinea pigs, blind mole-rats, naked mole-rats and humans. They exposed these tissues to chemicals that damage DNA and cause mutations. When exposed to the same dose of mutation-causing substances, the cells from a short-lived mouse quickly accumulated a lot of mutations. At the same time, there was hardly any mutation in the long-lived naked mole-rat or human. Thus there was some genetic mechanism helping long-lived animals to fend off cancer.
The study suggests that animals age as cells accumulate mutations. When nearly 3,200 mutations occur in its colonic epithelial stem cells, the mammal appears to reach the end of its lifespan. This does not mean with 3,201 mutations in a particular cell, the animal will drop dead from mutation overload. The relationship between mutations and ageing is a bit more nuanced. Nonetheless, the mechanism behind the mutations leading to ageing and death is still unclear.
One theory advanced by Cagan’s team tries to explain how the steady accumulation of mutations contributes to ageing. The explanation goes like this. As the animals age, all somatic cells pick up more and more mutations. While most mutations would be harmless or not vital, some mutations may occur in critical genes that regulate the cell’s behaviour in the tissue. Unchecked, these corrupted cells multiply and overtake the normal functional cells. With overwhelming malfunctioning cells, the organ hosting them fails, leading to disease and death.
Puzzle still remains
The recent advances in DNA sequencing technologies made these studies possible. Both the mutation count studies involved examining mutation in just one cell type, intestinal crypt cells or lung fibroblasts. If this result can be generalised to all somatic cells (meaning cells other than eggs and sperm) is a question that requires further studies. Keeping the limitations of both these studies, Cagan and his team propose a new study focusing on additional tissue types. Moreover, they are also expanding the samples from mammals, including other invertebrates and invertebrates. They plan to study tissue from the Greenland shark, which can live to over 400 years old and is the longest-living vertebrate in the world. They want to find if their results hold for the whole animal kingdom by covering a wide range of samples.
Peto’s paradox is only partially explained by the study. Longer the lifespan, the mutation clock ticks slow, thereby not accumulating more cancer-causing mutations compared to short-lived animals. However, the other part of the paradox, why big animals, with more cells, do not have high rates of cancer, still remains a mystery. Ancient Elephant Ancestor, Moeritherium, which lived about 55 million years ago, was just the size of a modern-day pig. If a giant modern elephant evolved from a small pig, most birds in their evolution become even smaller in size. How the evolution coped with the transition size of the animals and fine-tuned the mutations to control cancer incidence is yet another riddle. The search for an answer to Peto’s paradox goes on.
(The story first appeared on The Federal on June 20, 2022.)
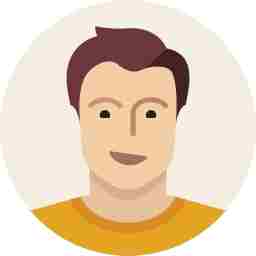