- Home
- IPL 2025
- The Great Language Divide
- News
- Premium
- THE FEDERAL SPECIAL
- Analysis
- States
- Perspective
- Videos
- Education
- Entertainment
- Elections
- Features
- Health
- Business
- Series
- Bishnoi's Men
- NEET TANGLE
- Economy Series
- Earth Day
- Kashmir’s Frozen Turbulence
- India@75
- The legend of Ramjanmabhoomi
- Liberalisation@30
- How to tame a dragon
- Celebrating biodiversity
- Farm Matters
- 50 days of solitude
- Bringing Migrants Home
- Budget 2020
- Jharkhand Votes
- The Federal Investigates
- The Federal Impact
- Vanishing Sand
- Gandhi @ 150
- Andhra Today
- Field report
- Operation Gulmarg
- Pandemic @1 Mn in India
- The Federal Year-End
- The Zero Year
- Science
- Brand studio
- Newsletter
- Elections 2024
- Home
- Delimitation Debate
- IPL 2025
- NewsNews
- Analysis
- StatesStates
- PerspectivePerspective
- VideosVideos
- Education
- Entertainment
- ElectionsElections
- Features
- Health
- BusinessBusiness
- Premium
- Loading...
Premium
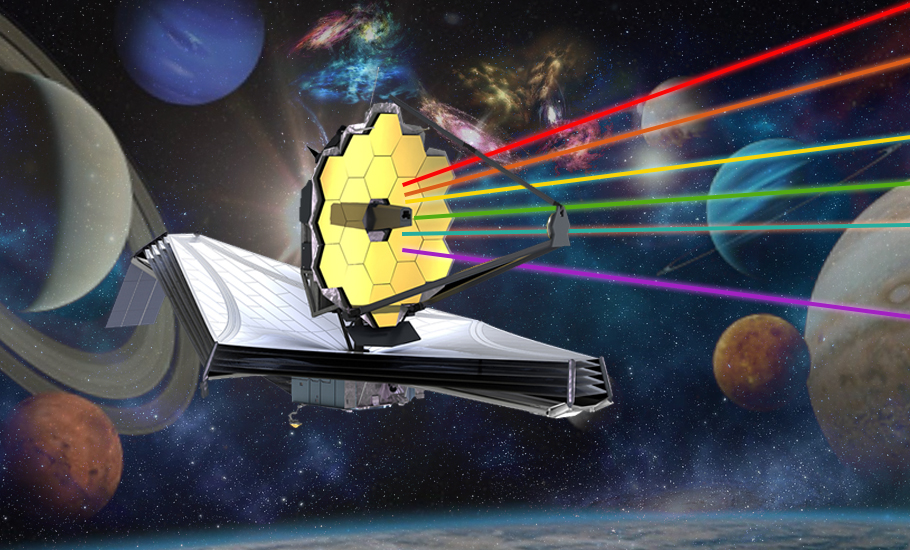
Cosmic mysteries that astronomers hope to solve with Webb Telescope
When Galilei Galileo famously turned his crude refracting telescope, with just 8x magnification, towards heaven on the night of August 25, 1609, the science of astronomy took a giant leap. Until then, only 1,022 stars catalogued in Almagest by Claudius Ptolemy (c. AD 100 – c. 170) were believed to exist in the whole universe. However, the handcrafted amateurish Galilean telescope...
When Galilei Galileo famously turned his crude refracting telescope, with just 8x magnification, towards heaven on the night of August 25, 1609, the science of astronomy took a giant leap. Until then, only 1,022 stars catalogued in Almagest by Claudius Ptolemy (c. AD 100 – c. 170) were believed to exist in the whole universe.
However, the handcrafted amateurish Galilean telescope showed hundreds of stars in every direction of the sky, so dim that it remained invisible to the naked eye. Black spots could be spotted on the Sun until then considered a symbol of perfection. Moon imagined to be smooth was dotted with craters and mountains. Jupiter was seen to be accompanied by four moons going around it. The phases of Venus seen from the telescope suggested that the planets are going around the Sun, and Earth is not the centre of the Universe. The upheaval caused by the petty telescope was so much that Galileo was demonised as a heretic, locked up in jail, and his books forbidden. If a crude instrument could do this much, imagine what a capable telescope can do?
Every new generation of telescopes brought new vistas. Planets Uranus, Neptune; many moons around Mars, Jupiter and Saturn; hundreds of solar system objects such as asteroids, exotic stars like neutron stars came to light. It also changed our perspective; Earth was not at the centre of the Universe; our Sun and our galaxy Milky Way are just one among the billions. While the night sky looks serene and placid, it appears cataclysmic through radio, X-ray and Gamma-ray telescopes, colliding galaxies, exploding stars and matter vanishing into the abyss of mysterious black holes.
What awaits JWST
With every new generation of telescopes, we get sweet surprises. In the anticipated 10 years of its lifetime, the JWST is sure to astonish us. The Hubble was able to get a hazy glimpse of the universe as it was 400 million years after the Big Bang. With six times the light-collecting area than the Hubble, the JWST must be able to see them clearly and show the universe as it was 100 million years after the Big Bang.
Billed as successor to the Hubble, the JSWT is expected to untangle many cosmic mysteries. While it is hard to predict what lurks hidden in the folds of deep space, astronomers pin their hopes to solve some of the mysteries of the universe. “During its first cycle, covering little more than a year, the JWST will spend 32 per cent of its time on galaxies, 23 per cent on exoplanets and 6 per cent on the Solar System. This will be the first science goal,” says prof Sujan Sengupta of Indian Institute of Astrophysics. “It can answer if the Solar System has a ninth planet. Using its powerful infrared eyes, a thorough survey beyond the Kuiper belt can answer if the Solar System has an Earth-sized, extremely faint and cool planet beyond the presently known last planet Neptune. If detected, it will be the last station of the Solar System,” he added. “I think exoplanet atmospheres and characterisation will be the most interesting results from the JWST,” says Dr Priya Hasan, assistant professor, Maulana Azad National Urdu University, Hyderabad.
How fast the universe is expanding
“One of the big enigmas in cosmology is the actual rate at which the universe is expanding, called Hubble constant (H0). Imagine that you measure your height first from head to toe and next from toe to head, you expect both measures to agree, but in the case of H0 measurement, they diverge. When you used the Planck satellite data on the cosmic microwave background radiation when the universe was just 3,80,000 years old, you get one value for H0. Alternatively, when astronomers measure the H0 with extreme precision using stars and galaxies from the late universe, we get another value. The difference is subtle but significant. Astronomers hope the JWST would be able to clarify this discrepancy — the so-called Hubble tension,” says Aniket Sule, associate professor, HBCSE (TIFR), Mumbai.
During the 1930s, using the powerful telescopes Edwin Hubble, an American astronomer, measured the distance and the velocities of galaxies. He plotted the graph of speed at which the object is directly moving away or towards the Earth, radial velocity, with the distance. The result was baffling — the farther the galaxies are, the faster they move. He proposed that the universe is expanding like the raisin bread dough expands inside the baking oven.
As the bread rises and expands, the raisins move farther away from each other, but they are still stuck in the dough. Furthermore, as seen from the viewpoint of any individual raisin, all the other raisins in the loaf appear to be receding away from each other. The nearby raisins move away slowly, and distant raisins move more quickly. Only one conclusion could be drawn — the universe is expanding like the raising loaf of bread.
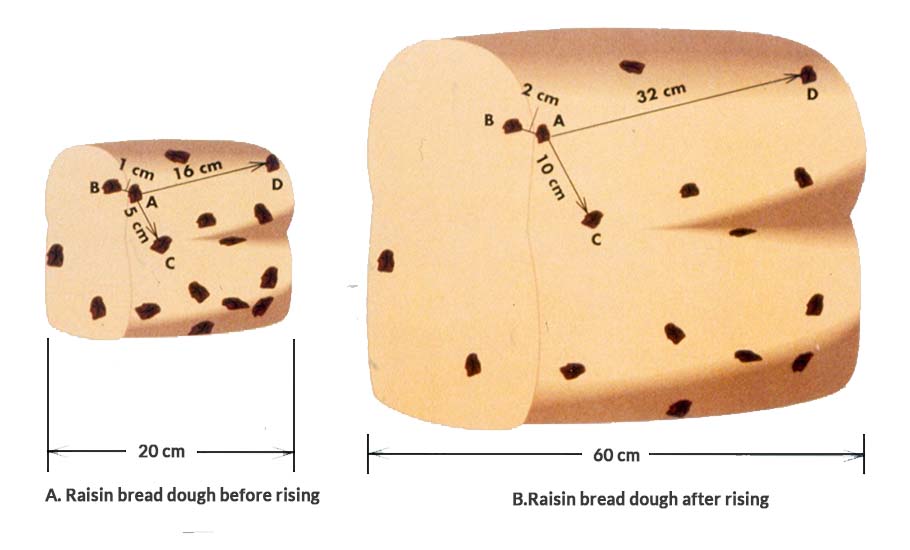
If we reverse the motion in time, about 13.8 billion years ago, cosmologists computed that all the stuff in the universe must have been concentrated at a single point. At that instant, the Big Bang, just in a flash, the cosmos mushroomed. So to say that was the beginning of the history of this universe.
The raisins are not exploding out inside the baking oven but are carried along by the expanding dough. Similarly, the Big Bang was not an ‘explosion’, but a sudden and continuous expansion of space-time. It is the growth of the fabric of space-time. As space-time expands, it carries the cluster of galaxies and all other cosmic objects.
There are three ways in which astronomers measure the rate at which the universe is expanding. The brightness of the 100 Watts on a bulb is accurately known. Suppose it is seen dim at a distance, we can measure its apparent brightness and compare it with its intrinsic brightness. The intensity of the light falls off at the rate of square of the distance; twice the distance intensity is one fourth. From this, we will be able to compute the distance of the bulb accurately. Likewise, some stellar objects aid astronomers to calculate the distance of faraway objects.
Typically astronomers use the Type 1a supernova in binaries with one typical star waltzing around a super-dense white dwarf star. The white dwarf siphons off matter from the companion. A runaway thermonuclear explosion rips through a white dwarf star and blows the star to bits when sufficient mass builds up. The intensity of such an explosion outshines an entire galaxy with billions of stars and is known precisely. Astronomers can spot them billions of light-years away.
“From the physics, we squarely know the brightness of the Type 1a supernova. By comparing the brightness with which it appears in our telescope and its intrinsic brightness, we can compute the distance of the galaxy in which the supernova occurs. We can measure how fast the galaxy is moving away from us. Dividing the speed by the distance, we get the rate; value of H0,” says Sule. In addition to Type 1a supernova, other stars, such as Cepheids, RR Lyrae, certain types of giant stars and so on, with known internal brightness, serve as ‘standard candles’. They are sources of light that do not vary from one particular instance to another. Hence, their apparent brightness tells us its relative distance. We can compute how far they are situated by looking at them in distant galaxies.
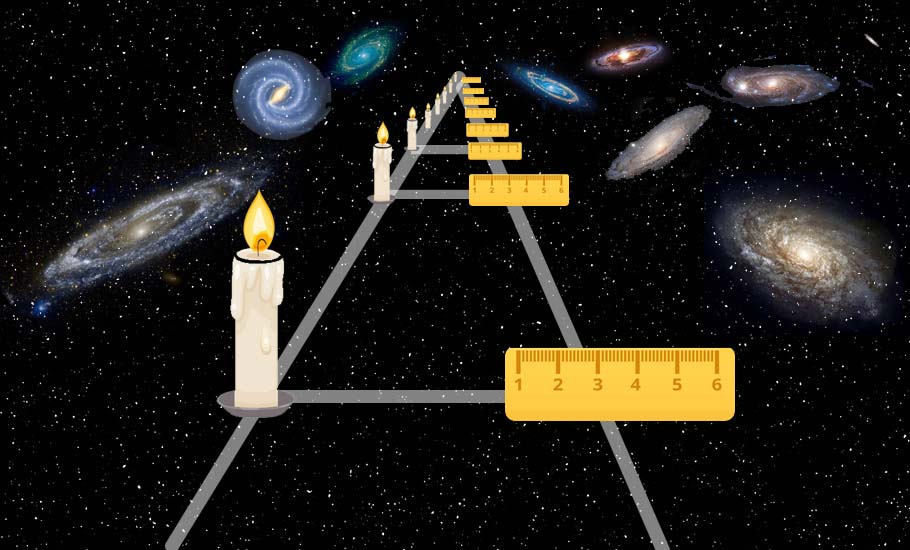
“The second method to compute the H0 is to compare the unevenness found in the Cosmic Microwave Background Radiation (CMBR). We use this graininess as ‘standard rulers’ and measure the growth and expansion of the large-scale structure to the present day,” says Dr Sule.
At the time of the Big Bang, the universe was hot, dense and uniform. As the universe expanded, it cooled. As it cooled enough, the formless matter evolved into fundamental particles like quarks, which aggregated to form subatomic particles such as protons, neutrons and the like. The subatomic particles nucleated and formed the atoms, mostly hydrogen peppered with small quantities of helium and trace amounts of lithium. The atoms collated to form galaxies, stars and planets. The universe, as we know it, slowly evolved.
A particular instance in the history of the universe, when it was 3,79,000 years old, is unique. At that time, the whole universe was filled with the hot dense plasma of hydrogen, helium mixed with a dash of lithium. The temperature of the universe was about 3,000 Kelvin. At that temperature, the atoms were excited, and they released photons before reaching the ground state. These primordial light photons from this era of the universe are still visible to us; they bombard us from all sides.
The universe has cooled down to 2.7255 K today. After journeying through the expanding universe for some 13.8 billion years, the leftover light from the early universe has redshifted so much that it now appears in the microwave portion of the spectrum. Called cosmic microwave background radiation (CMBR), this is the oldest observation we currently have of the universe.
Like the footprint on sand, the CMBR holds the imprint of the tiny weeny clumpy density differences in that stage of the universe. At that time, the universe was just 3,79,000 years old; therefore, light could have travelled at best a distance of 3,79,000 light-years. Hence the minute clumps in the early plasma soup cannot be more than 3,79,000 light-years across. These spots grew into the large-scale structure in the universe, galaxies, clusters of galaxies and superclusters of galaxies we study today.
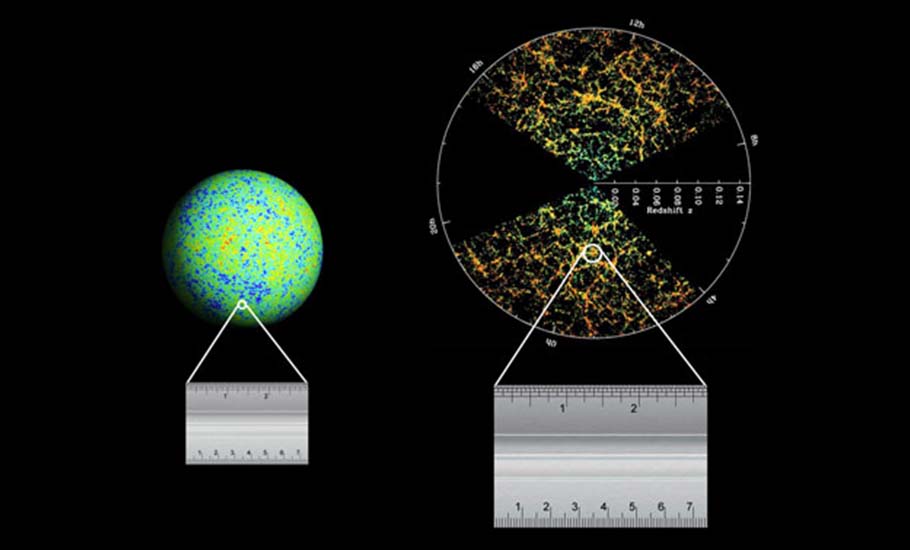
The clumps in the CMB are like the distance between the raisins in the bread dough. The actual size of large scale structures we observe today is like the distance between the raisins after the bread is baked. By comparing both, we can know how much the universe has expanded in the past 13.8 billion years. From this, we can obtain the value of H0.
In the first method, we begin from today and work backwards towards the universe’s infancy. In the second, we commence measurement from the earliest states and work forward until today to compute the value of H0.
“Measuring the H0 from the standard candle is like measuring your height from head to toe; measuring the H0 from the CMB clumps is like measuring your height from toe to head. We would expect them to be equal. Alas, there is a difference; the discrepancy is subtle. However, it still matters a lot,” says Sule. The value of H0 from Plank data is about 67. This means the expansion rate is 67 kilometres per second faster every 3.26 million light-years. However, the value derived from the supernova measurement is around 74. Gravitational-wave astronomy also provides an alternative method of measuring the H0. Sadly, it too is inadequate to untangle the mess.
With its eagle eye, the JWST will be able to possibly observe some of the early Type I supernova explosions and other standard candles and help us better measure the rate of the expansion of the universe. “Measuring the value of the Hubble parameter at the time of the earliest galaxies will help us resolve the ‘Hubble tension,’ that is, the discrepancy in the value of the Hubble parameter in the nearby universe and CMBR,” says Sule.
How and when the first galaxies formed
“I am interested in the investigation of how the first galaxies formed,” says Prof Somak Raychaudhury, director, Inter-University Centre for Astronomy and Astrophysics, Pune. “The epoch of galaxy formation was an early chapter in the evolution of the universe. Until now, we lacked the capacity to observe them in the first place,” he added.
We know that there were no stars or galaxies at the time of the Big Bang. In the course of the evolution of the universe, the ‘first star’ and the ‘first galaxy’ must have formed somewhere back in time. The youngest galaxy we have seen so far, Gz-11, is from when the universe was 400 mere million years old. There should have been stars and galaxies before that. The Hubble and the Spitzer space telescopes are not powerful enough to look deep into the past.
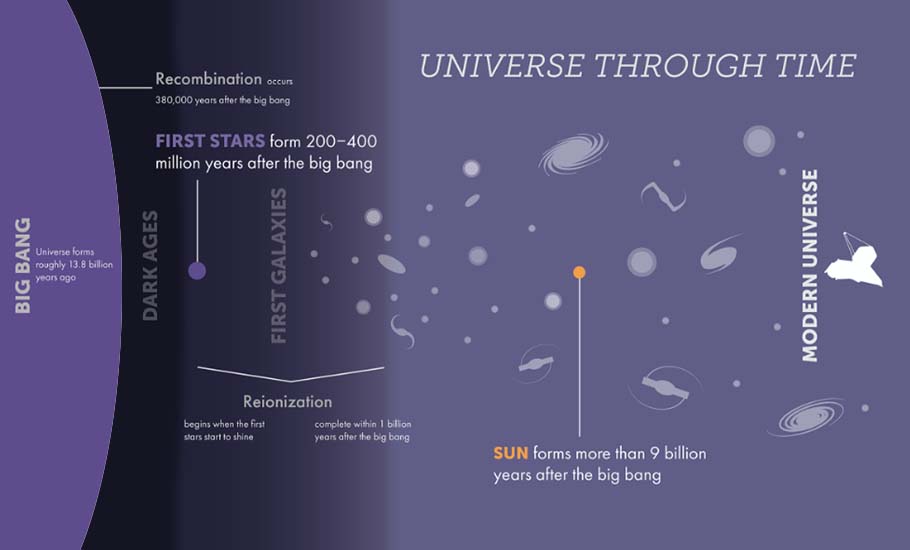
“If we want to observe the past of the universe, we have to look very far away because light takes time to travel,” says prof Raychaudhury. However, as the ancient starlight from the infant galaxies traversed through the expanding fabric of space for billions of years, the waves became stretched. “The light from galaxies from when the Universe was 1/8th of its current age will be redshifted by a factor of 9,” points Prof Raychaudhury.
Redshift is an example of the Doppler Effect. The siren of an ambulance is higher in pitch when it is approaching than when it is receding. Called the Doppler Effect, this effect is observed whenever the wave source moves relative to an observer. As the universe is expanding, light from the moving objects shifts to the red end of the spectrum as its wavelengths are stretched to longer (or redder) wavelengths.
Redshift 9 means that the wavelength of the rays as they traversed through the expanding space has stretched nine times. “Therefore, while the nearby galaxies appear blue, ancient starlight from infant galaxies would be at near-infrared,” explains Prof Raychaudhury.
In short, the 0.4-micron wavelength blue and ultraviolet radiation from the early young stars and galaxies would elongate, travelling billions of years to become 0.4 x 9 = 3.6-micron wavelength in the infrared region. “The Hubble telescope is sensitive to wavelength 0.1-1.8 microns, therefore, enabling us to see the nearby galaxies in UV vision. However, suppose we want to see the infant galaxies from billions of years ago. In that case, we need an infrared telescope, which is what the JWST provides us,” he adds.
Scanning the cosmic womb
“A truly exciting area in which the JWST will excel is that of star and planet formation,” says Dr Niruj Ramanujam from the Indian Institute of Astrophysics, Bengaluru. Recent discoveries of extrasolar planets or exoplanets have challenged our conventional wisdom on the evolution of the planetary system. Like the Solar System, we presumed the other planetary systems would have rocky planets such as Venus and Earth closer to the host star. Gas giants and icy planets will follow this in that order. This would be the norm throughout the universe, so we thought. However, many observed exo-planets are ‘hot Jupiter’ types. They are ‘Jupiter-sized’ and orbit closer than Mercury to the host star. Our solar system seems to be an outlier among the thousands of planetary systems discovered.
Ground-based telescopes and the Hubble have been able to identify the stellar nurseries where stars and their planetary systems take birth. The stars and their planets form when clumps of gas and dust contract. Hence, typically, these protoplanetary systems are shrouded in an envelope of dense dust clouds.
“These stellar nurseries are shrouded in dust, which is opaque to visible light, and will hence block our view,” says Niraj. While the visible light is blocked by the dust surrounding protoplanetary systems, they are transparent in infrared rays. “The mid-infrared instruments aboard the JWST can peer into extremely dense dust clouds,” says Niruj, adding, “The telescopes are kept at 7 Kelvin, or -266 deg Celsius. Such low temperatures are needed so that the infrared emission from the JWST itself does not overpower the cosmic signals.” Ultrasound images help us see the development of the fetus. The JWST would uncover the veil around the stellar nurseries and show us the process of the evolution of planetary systems.
Hello, anyone out there?
“The JWST is the best hope for us to answer within the current decade, mankind’s eternal question: ‘Are We Alone?'” says prof Sujan Sengupta of the Indian Institute of Astrophysics. “The JWST is capable of not only detecting habitable exoplanets around stars far away from us but can also probe their atmosphere rigorously and detect bio-signature in the infrared wavelengths,” he adds.
When light, including infrared light, passes through gases, specific wavelengths are absorbed depending upon the chemical composition. The ‘transmission’ spectra will lack or show a decrease in strength in these wavelengths. Appearing as dark absorption lines, like a fingerprint, these indicate the elemental composition of the absorbing gases.
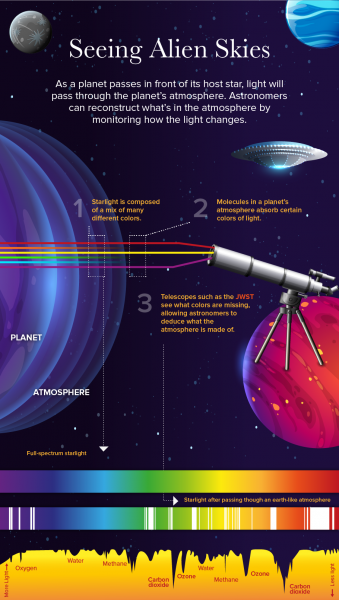
Suppose, there is an exoplanet with an atmosphere and its orbit is so tilted that it transits the host star as seen from Earth. When the host star, exoplanet and Earth are in a straight line, the starlight will dim, due to the obstruction, clearly indicating the presence of a planet. Further, part of the starlight will pass through the atmosphere of the exoplanet. Depending upon the composition of the exoplanet’s atmosphere, some wavelengths of spectra will now show dark absorption lines. These ‘transmission spectra’ can be compared with the star’s spectra when the planet is not in transit. We can infer the elemental composition of the exoplanet’s atmosphere.
“The JWST will take ‘transmission spectra’ by using MIRI (Mid InfraRed Instrument) during the transit of the planet (when the planet is between the star and the telescope). For a habitable planet around a Solar type star, it would take a year for each transit event,” says prof Sengupta. Using the infrared spectroscopy we will be looking for oxygen, ozone, methane, phosphine and so on in addition to water by taking the spectra of starlight that passes through the atmosphere of the planet during its transit across the star, he adds.
Methane on Earth is primarily produced by cows but is abundant on Jupiter, Saturn, Uranus and Neptune. The presence of one chemical is not enough to be sure of its biological origin. Nevertheless, a specific concoction of potential biomolecules would indicate a possible biological source. “On Jupiter, Saturn etc., methane is produced chemically as these planets are hydrogen-rich. But it cannot survive in the presence of oxygen. The continuous biosynthesis of methane causes chemical imbalance in Earth, and we find excess methane. Thus, in an oxygenic atmosphere, methane serves as potential biosignature,” explains Prof Sengupta.
So, the JWST may perhaps help find a planet with life beyond Earth.
(This is the second article in a two-part series on the James Webb Space Telescope. You can read the first article here.)
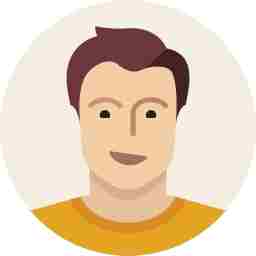