- Home
- News
- Analysis
- States
- Perspective
- Videos
- Education
- Entertainment
- Elections
- Sports
- Features
- Health
- Budget 2024-25
- Business
- Series
- Bishnoi's Men
- NEET TANGLE
- Economy Series
- Earth Day
- Kashmir’s Frozen Turbulence
- India@75
- The legend of Ramjanmabhoomi
- Liberalisation@30
- How to tame a dragon
- Celebrating biodiversity
- Farm Matters
- 50 days of solitude
- Bringing Migrants Home
- Budget 2020
- Jharkhand Votes
- The Federal Investigates
- The Federal Impact
- Vanishing Sand
- Gandhi @ 150
- Andhra Today
- Field report
- Operation Gulmarg
- Pandemic @1 Mn in India
- The Federal Year-End
- The Zero Year
- Premium
- Science
- Brand studio
- Newsletter
- Elections 2024
- Home
- NewsNews
- Analysis
- StatesStates
- PerspectivePerspective
- VideosVideos
- Entertainment
- ElectionsElections
- Sports
- Features
- BusinessBusiness
- Premium
- Loading...
Premium - Elections 2024
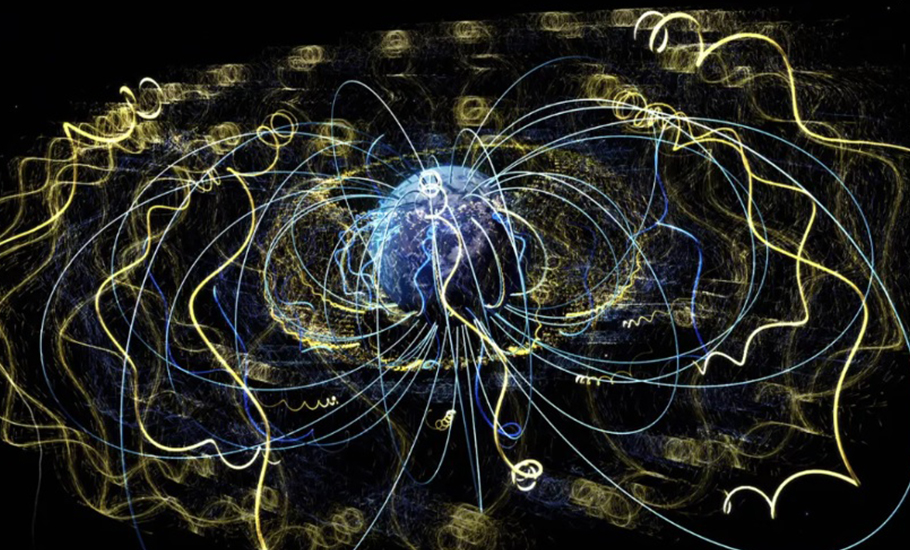
How living magnets could sound the death knell for cancer cells
Many animals have an innate sense to detect the earth’s magnetic field, which they use for navigation. It’s fascinating to know that certain bacteria, called magnetotactic bacteria, go a step further: they make magnetic compasses in their cells. The magnetic compasses are called magnetosomes and are nano-sized crystals of magnetic material (nano is 1 x 10-6 mm). The bacteria employ...
Many animals have an innate sense to detect the earth’s magnetic field, which they use for navigation. It’s fascinating to know that certain bacteria, called magnetotactic bacteria, go a step further: they make magnetic compasses in their cells.
The magnetic compasses are called magnetosomes and are nano-sized crystals of magnetic material (nano is 1 x 10-6 mm). The bacteria employ complex cell processes to make magnetosomes: first, iron is absorbed from their surroundings. The metal is then converted into 25-100 nm-sized crystals of iron oxides or iron sulfides — these minerals have magnetic properties. The nanocrystal is then wrapped in a protective layer of lipids (fats).
The completed package is called a magnetosome which acts like a tiny magnet with a north-south pole. The bacteria synthesise many magnetosomes in their cell and string them together. The chain of magnetosomes behaves like a magnetic compass and aligns parallel to the earth’s magnetic field lines. As a result, magnetic torque develops in the cell.
The bacterium uses the torque to push its tail-like extension called a flagellum. The movement of the flagellum, in turn, steers the organism in its surroundings.
Magnetotactic bacteria are aquatic and anaerobic organisms. They do not need oxygen for cell functions and hence thrive in regions with an oxygen gradient. So these organisms are often found swimming from high oxygen to low oxygen regions in the water bodies.
Researchers have found that magnetosomes are highly stable nanoparticles which retain their stability even when isolated from the bacterial cell. Owing to this and many other properties established by recent experiments, scientists are actively exploring ways to use the bacteria and the magnetosomes as nanobiots – nano biological robots for medical purposes.
New-age cancer therapies
Currently, chemotherapy and radiation are the methods to treat cancer. However, they have many limitations. High doses of oral or intravenous medications cause severe side effects and can be toxic to other organs. Although radiation is given at the tumour site, some parts of the surrounding healthy tissue are often damaged. In addition, detecting tumours in their early stages and marking the tumour boundary (at the cell level) is challenging. It depends on the high contrast accuracy of the imaging equipment, such as MRI (magnetic resonance imaging).
Experiments show that magnetotactic bacteria and magnetosomes can come to the rescue in overcoming these challenges. Furthermore, they offer a targeted and localised treatment option.
Targeting cancer cells
It is well known that cancer cells are devoid of oxygen. As magnetotactic bacteria seek low oxygen environments, scientists propose that using these bacteria can help accurately target cancer cells. In recent experiments, scientists injected magnetotactic bacteria in diseased mice and observed that the bacteria move towards the cancerous region.
High contrast imaging
In others studies, researchers injected the bacteria in dead mice and tracked the tumour using MRI. They found that the presence of magnetosomes offered a better image contrast, from which they could observe the tumour boundary with more clarity. In their experiments, they discovered that smaller-sized magnetosomes of 25nm size gave a better image contrast compared to bigger crystals.
Inducing hyperthermia
Research has also shown that magnetosomes are viable candidates to induce hyperthermia or excess heat in cancer cells and destroy them. When magnetosomes are exposed to an alternating magnetic field, they respond in two ways: i) rapidly change their magnetic moment (direction of poles) and ii) physically rotate to align with the field. Both actions expend energy that is dissipated as heat. Experiments have shown that hyperthermia raises the surrounding temperature by 5-7 degrees. When localised in the microenvironment of the cells, this temperature density is enough to induce cell apoptosis (a type of programmed cell death) in cancer cells. Further experiments show that a chain of magnetosomes induces better temperatures.
Drug delivery
Bacterial magnetosomes are naturally synthesised nanoparticles. Their outer protective layer is like the exterior of a cell and hence can be manipulated. Scientists are experimenting with isolated magnetosomes by modifying their outer layer, loading it with cancer-specific drug molecules, and then re-covering it with a protective coating. When these particles reach the tumour region, the high acidic environment of cancer cells degrades the protective cover, exposing the drug. This way, they can directly deliver drugs to cancer cells to destroy them. Such a scheme requires low drug doses and drastically avoids side effects.
Other uses of magnetosomes
Studies indicate magnetosomes are highly stable and magnetic under physiological (body) conditions. Hence they are useful in various other medical applications. For example, magnetosomes can be used to diagnose and monitor diseases such as diabetes and cancer. Furthermore, when used in immunoassays, they can detect pollutants or specific molecules and for cell division and DNA extraction. In all these areas, the magnetosome outer layer is modified with a particular molecule or antibody for detection.
Nothing beats natural
There are still several unknowns about how magnetotactic bacteria synthesise magnetosomes. Hence making artificial magnetosomes is beyond our current capability. However, scientists are attempting to make chemically derived magnetic nanoparticles (MNPs). But they have several drawbacks:
• The size of MNPs is crucial as they have to pass unhindered through the body’s blood and brain barrier (BBB). The BBB is the body’s natural filter mechanism – a safety net of tissue – that arrests the entry of unwanted or toxic elements into the system.
• Therefore, designing ultra-small MNPs (10-50nm) is a huge challenge as the particles become highly unstable and do not have a uniform crystalline structure.
• Although non-toxic MNPs can be designed, the making process involves toxic chemicals and procedures. Therefore, though several potential MNPs are proposed, only one iron oxide MNP has been approved for clinical trials so far.
Research shows that natural magnetosomes derived from bacteria score better than these synthetic molecules on several factors.
• The bacteria are non-pathogenic (do not cause diseases in humans), and the magnetosomes are non-toxic.
• The magnetosome crystals are highly uniform and stable in body fluids
• They have high and stable magnetic properties allowing for manipulating them with external fields
Using magnetotactic bacteria as therapeutic tools is highly promising; however, current ethical mandates do not allow using them in clinical trials. Hence, scientists propose an alternative: to make the best use of magnetosomes produced by these bacteria.
Presently, among the various known magnetotactic bacteria, only magnetospirillum gryphiswaldense is found to be most suitable for medical applications. Research has shown that the species has a fast growth rate and can be grown using non-toxic growth media and methods. Furthermore, under optimum growth conditions, the bacteria have a high magnetosome yield (reportedly, 170mg/litre/day ). Further, scientists have studied the bacteria’s genetic makeup and can manipulate them to yield magnetosomes of required sizes.
Fast forward
In the 1950s, Dr Richard Feynman, the visionary scientist, spoke ardently about miniaturisation. He envisioned that in future there was the possibility that we could inject objects smaller than cells into our veins and manoeuvre the tiny bots at that level.
His vision could fast become a reality with magnetosomes.