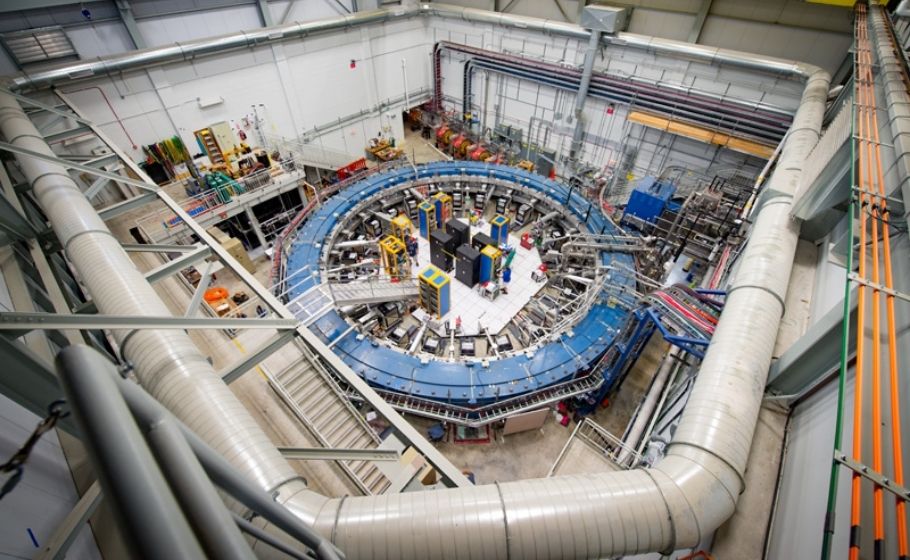
Who ordered muon? Physicists awaiting a Higgs boson-like discovery
Muon is the sibling of electron. Discovered by Carl Anderson and Seth Neddermeyer in 1936 while studying cosmic rays, it looks and behaves exactly like electron but heavier. It has the charge of electron and spin is also half.
Muon is the sibling of electron. Discovered by Carl Anderson and Seth Neddermeyer in 1936 while studying cosmic rays, it looks and behaves exactly like electron but heavier. It has the charge of electron and spin is also half. But the mass is 206.7 times heavier than electron. It has a lifetime of 2.2 microseconds when it is at rest. But it travels close to the speed of light it can live much longer, thanks to Einstein’s relativity.
Like other elementary particles, it also has an antiparticle of exactly identical mass. It was an unexpected discovery and mysterious that Nobel laureate Rabi wondered “who ordered muon?” It is denoted by the Greek symbol µ−. The negative sign indicates the charge.
The standard model of Particle Physics formulated in 1971 has 17 particles. Muon has become one of the center of attraction since physicists hope it will help in understanding what is beyond? The understanding and control on handling of the muons have become so good that even it has become commercially useful. Since they penetrate more than X rays and Gamma rays, they are used as muon tomography to scan huge containers for nuclear material and contrabands like the body x-ray machines at the airports! They have been used to find the hidden chambers of pyramids. Fukushima nuclear cores were mapped using muon beams!
Going back to high energy physics, muons are attractive since they are very light and hence they can be produced enormously. But they are sufficiently heavy, unlike electrons, to help us experiment to understand how we can go beyond the standard model. This is what is driving many in the Particle Physics community internationally to come together.
Research centers like Brookhaven National Laboratory, Fermi Lab as well as from Switzerland and Japan, have joined together to study and understand muon. Belief is that this will give us knowledge of the sub nuclear physics.
Muons are probes to see why there are so many families of elementary particles. What is the symmetry beyond what we know at present? How do we classify them? One of the important common property of electrons and muons as expected from quantum theory is their value of the magnetic moment which is two times a unit called Bohr Magneton. That is each muon is a magnet. This is denoted by g. So we expect g-2 to be 0. But the underlying interactions modify it by a tiny amount which is known as anomalous magnetic moment. This happens for electrons as well as muons. The reason behind this: magnetic moment is contributed by the spin which wobbles in magnetic field like a top. But the top wobbles differently on a smooth glass or tiles or rough stone. Environment provides the difference. This is measured by a number called ae which is half of g-2 for electron. This has been measured accurately and agrees to 10 decimal places.
Related news |Â Remembering Raman: Physicist who demystified science, taught by example
This has been calculated for muon and experimentally verified to few decimal places. But the environment for muon being different changes the value. To compare with experiment we need first to understand what is the background in which muon wobbles. Standard model gives an understanding of that. This is modified due to the nature of interactions of muon, like elctric charge, weak and strong interactions.
There are detailed calculations performed by hundreds of experts and the contribution has been completely analysed in three parts. This theoretical calculation itself was an international effort involving approximately 130 scientists from 78 Institutions in 21 countries. They computed, checked, and made independent tests and finally concluded:
aµ = aQED + aEW + aQCD = .00116591810
The above is the anomalous magnetic moment of the muon.
We can see a small difference from seventh decimal place onwards. This discrepancy is with 95th question physicists would want to settle is whether this difference is real and if so what we can infer from that.
A new experiment called muon g-2, improving the accuracy of the number given earlier has been in progress from 2018 at Fermi Lab. This will end in 2022. Enormous amount of data have been collected and analysis of data for the first year has been completed and will be announced on April 7, 2021, at 10AM EST.
Related news |Â 3 scientists win Nobel Prize in Physics for discoveries in cosmology
What was done at Fermi Lab? About 10 trillion protons (mainly nucleus of hydrogen atom, and hence ionised hydrogen) are smashed into a target which produce a large number pions. These pions are the glue which hold the nucleus together.
They eventually decay to muons. These muons travelling at the speed of light are guided through powerful magnetic field. These muons through their magnets attached to them through their spin wobble like a top. This wobbling depends as mentioned on the background and the background is what one wants to find. These muons decay producing neutrinos which fly away along with positrons. These positrons provide the information about the magnetic moment of the muons precisely and hence about the background to them. Already we can see how complex the experimental arrangements are. The accuracy is expected to be about 140 parts per billion.
High energy physics community is anxiously waiting whether the data confirms, improves the deviation from the standard model prediction.
This will tell us whether the background in which the muons live, have additional new particles which are not accounted in the standard model.
It can give us directions for further physics beyond what we know and hold as sacred. If it is so, it will be extraordinarily exciting for the physicists. To compare, it will be like the discovery of Higgs boson which was the missing link for the standard model.