Galloping horses and waltz of electrons: 2023 Physics Nobel explained
What Pierre Agostini, Ferenc Krausz and Anne L'Huillier developed, and how it can help humankind
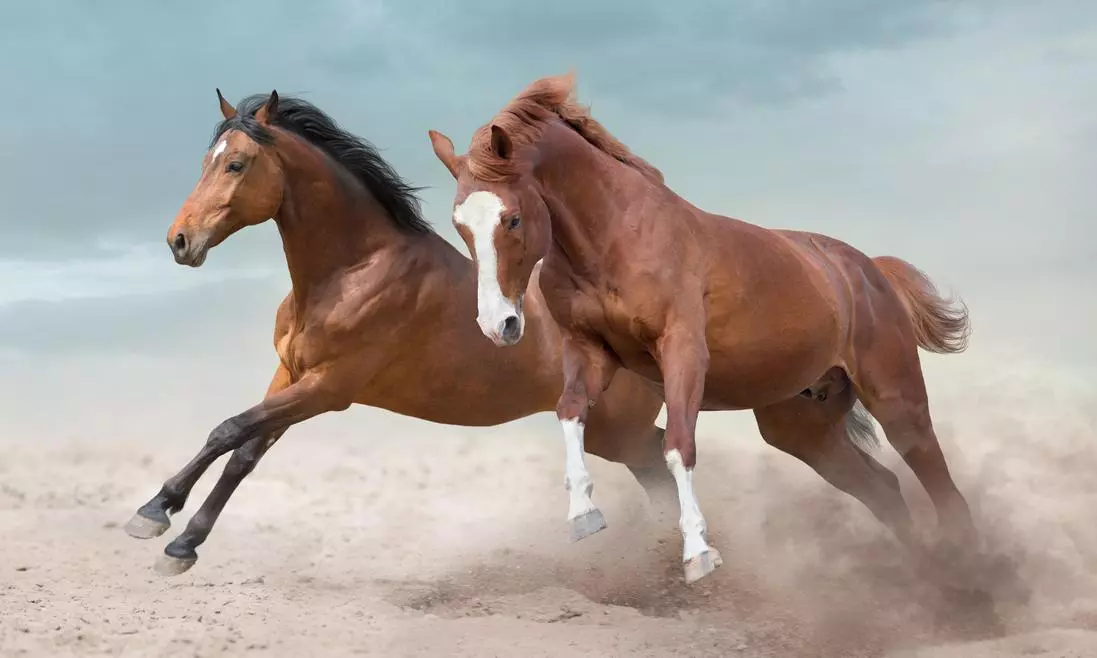
Horses have captured our imagination from ancient times. Horses have been part of artistic repertoire from the prehistoric caves of Bhimbetka rock shelters near Bhopal to modern times.Images and sculptures of the galloping horse are often depicted with its hind legs gathered under the stomach, with the front legs left to “paw the air” off the ground.
When the horse galloped, did all four hooves leave the earth, momentarily making the horse airborne?
Enigma of horse gait
Lenon Stanford, an American millionaire philanthropist (who would donate funds to establish Stanford University as a memorial of his only son lost to typhoid), had the money and interest to wonder about this.
The movement of the galloping legs was too fast for observation with the naked eye. It was clear only a photograph could capture the stages of gait and prove that the horse has all four feet in the air during some parts of its stride. Alas, the photographs required nearly 15 seconds to one minute exposure time, unfit to capture the rapid movement of the galloping horse.
In 1878, photographer Eadweard Muybridge came up with an ingenious solution. Financed liberally by Stanford, he developed more sensitive emulsions. Muybridge then installed a battery of 12 cameras evenly spaced. Each of the cameras was linked to a trip wire. The horse pulled a two-wheeled carriage and galloped; the wheels pushed the wire, triggering the switch opening the camera’s shutter. As the horse jogged forward, the wire tripped, and one by one, the 12 cameras were set off in rapid succession in less than half a second. The photographic plates were developed, and all four feet were seen tucked under the horse, contrary to the artist's imagination.
What about electrons in an atom?
Around the same time, in 1887, German physicist Heinrich Rudolf Hertz discovered what came to be known as the photoelectric effect. German physicist Philipp Lenard clarified that electrically charged particles, electrons, are liberated from a metal surface when illuminated by ultraviolet rays.
Einstein explained the phenomenon and got the Nobel Prize. Einstein said that the photon, the particle of light with the right energy, rips an electron off the metallic atom. The energy of the photon is transferred to the electron. The electron with higher energy can free itself from the atom's grasp and stream out. The same phenomenon makes the solar panel produce electricity with sunlight.
When the light strikes, is the ejection of electrons instantaneous? To reasonably capture all stages of the gait of a galloping horse, we need a camera that can shoot images at a hundredth of a second. To capture the movement of an electron inside an atom, we need cameras that can take pictures at hundreds of attoseconds (attosecond = 0.000000000000000001 of a second). Werner Heisenberg, one of the pioneers of quantum mechanics, 1925, opined that feasibility is in the realm of the impossible.
Earth takes a year to go around the Sun, while Neptune takes 165 years. To measure heartbeat and blinking of an eye, we use minutes and seconds. Packet switching for internet communication requires measurement of time with milliseconds accuracy. Atoms and molecules are tiny, typically between 0.1 to 0.3 nanometres. A flash of light will take an attosecond to travel a distance of 0.3 nm. To capture events at an attosecond timescale and figure out the dance of electrons resulting in molecular bonds and chemical reactions, we need to create light pulses that are attosecond, that is, one billionth of a nanosecond; Heisenberg thought that was impossible.
Quest for a superfast camera
Just as Muybridge needed the right contraption to capture the gait of the galloping horse, physicists needed a superfast camera to capture the movement of the electrons. When the object is moving fast, the images are blurred. However, with fast strobe light to illuminate the thing for a fraction of a second, we can take pictures like it has been frozen in time. One needs a flash of light that is attracted long to capture the movement of electrons inside atoms and molecules. The story of the physics Nobel Prize to Pierre Agostini, Ferenc Krausz, and Anne L'Huillier is the story of the invention of the superfast camera that could capture the nimble movement of electrons in atoms and molecules.
The story begins with Ahmed Hassan Zewail (February 26, 1946 – August 2, 2016), an Egyptian and American chemist who innovated strobe light that flashes just tens and hundreds of femtoseconds (one-millionth of one billionth of a second) in duration. In his pioneering study, Zewail showed that reactions between hydrogen atoms and carbon dioxide: H + CO2 → CO + OH have an intermediate stage. HOCO is formed and lasts for 1000 femtoseconds before it disintegrates into CO + OH. He was awarded the Nobel Prize in 1999 for establishing femtochemistry, the study of chemical reactions that take place in tens and hundreds of femtoseconds.
Even with the best strobe effect, we can obtain only a pulse of femtoseconds, adequate for understanding reactions between molecules. However, we need a thousand times smaller pules than a femtosecond to image the reactions inside atoms and molecules.
The wavelength of visible light ranges between 400 nanometres (violet light) to 700 nanometres (red light). A single wave will take between 1.3 and 2.3 femtoseconds to go from crest to trough to crest again. Infinitesimal pulses are still too immense to capture the waltz of the electrons. The problem appeared to be insurmountable.
Out of box thinking
Can you measure units that are smaller than the markings on the scale? Well, it depends. Suppose we have only a 5-litre and 3-litre measuring jar; still, we can measure one litre of water with ingenuity.
Fill the 3-litre can with water.
Pour the water from the 3-litre can into the 5-litre can.
Fill the 3-litre can again with water.
Pour the water from the 3-litre can into the 5-litre can until it is complete.
Lo behold, we are left with 1 litre of water in the 3 litre can.
Anne L'Huillier and her team came up with a somewhat similar solution.
Light is also a wave, which means it has a crest and trough.
When two waves meet, exciting things happen. Suppose the wavelength is exact and the crest of one is aligned precisely with another. In that case, they add up and the resultant wave amplitude increases.
However, if the wavelength is identical and the crest of one exactly meets the trough of the other's, they cancel out.
When two waves with different wavelengths meet, they create a hybrid wave, which is super-positioned.
In 1987, Anne L’Huillier and her collaborators made an intriguing observation: like a plucked string instrument can produce overtones, atoms of certain noble gases excited with powerful infrared lasers can produce ultraviolet light that oscillates multiple times faster than the original laser.
She and her colleagues found that the incident laser yanked an electron from the gas atom. However, within a short duration, the electron returned to the atom, beaming a pulse of light. While doing so, the resultant waves were harmonic overtones. The overtones were not just two or three times the frequency but even as high as thirty times. That is, the overtones' oscillation was much faster than the incident light. After much exploration, they could precisely compute the resultant overtone's frequencies. Later, they worked out ways to overlay them, so they added up to a new hybrid wave.
Combining bits and pieces of music from various instruments in an orchestra, one can ensemble a symphony. L'Huillier and her team found that by combining light of different wavelengths, we can get the pulse of light of the desired duration. After years of work, in the 1990s, L'Huillier and colleagues demonstrated that they could combine different waves to interfere, producing shorter pulses where the peaks coincide. The new hybrid wave was like a pulse with a crest or through interspersed with destructive interference of a specific duration.
This marked the birth of attosecond physics.
Enter Pierre Agostini. In 2001, Agostini and his team could combine the trains so that consecutive light pulses flashed at an interval of just 250 attoseconds. Meanwhile, Ferenc Krausz and his team were able to isolate a single light pulse that lasted 650 attoseconds. The race for the fastest flash ensued. In 2001, Agostini's team flashed a pulse that lasted just 250 attoseconds. Soon, in 2003, L'Huillier's group came up with a twinkle that was just 170 attoseconds long. Ferenc Krausz, in 2008, achieved pulses that were just 80-attosecond. Today, Hans Jakob Woerner's group has managed a pulse of just 43 attoseconds.
What can you do with attosecond pulses?
Like a high-speed camera could capture a horse's gait with an attosecond pulse of light, physicists can understand the dynamics of electrons in atoms and molecules. Attosecond laser provides a ‘movie’ of the dance of electrons inside atoms and molecules. By 2008, Krausz could generate a pulse that flashed for 80 attoseconds. With this, he and his team could show that during the photoelectric effect, the ejection of the electron was not instantaneous. Neon atoms have two electrons in their first shell and eight in the outermost shell. Striking with the 100-electron volt light pulse, they found that an electron in the lower-energy state is ejected 21 attoseconds faster than one in a higher-energy state. Later, another experiment conducted in 2020 showed that electrons escape tens of attoseconds faster from liquid water than from water vapour.
In a semiconductor induced by light, the electron jumps the band gap. Recently, scientists have been able to take snapshots of this phenomenon using attosecond pulses of soft X-ray light and proved that it takes around 450 attoseconds for the electron to hop across.
Scientists have used the new tool to measure the time to oust an electron bound inside an atom. This measure indicates how tightly the electron is held within the atom in the first place. They have also been able to take snapshots and combine them to make a ‘movie’ of how electrons move from place to place inside molecules.
Physicists have also demonstrated that an attosecond pulse can be an ultrafast light-induced switch. Shine the pulse electrons are ejected, making the material an ionic conductor. Switch off the pulse, the electrons return, and the material remains insulator.
Krausz and his team are experimenting with biological samples and have recently shown that attosecond flashes on human blood induce changes that differ in normal and cancerous cells.
(The author is a scientist with Vigyan Prasar, New Delhi)